By Titus Szobody
The exploration of space has always been at the forefront of human curiosity and scientific advancement. One critical aspect of space exploration is our ability to observe and understand the universe beyond our atmosphere. Telescopes play a pivotal role in this endeavor, allowing us to peer into the depths of space. As technology evolves, researchers are constantly pushing the boundaries to enhance the capabilities of space telescopes.
Traditional telescopes often employ large, solid mirrors to capture and focus incoming light. The most powerful and advanced space telescope to date is NASA’s James Webb Space Telescope, which utilizes a primary mirror with an unprecedented diameter of 6.5 meters. While these mirrors have proven effective, they are also heavy and challenging to fit on a payload for launch and position in space. In addition, these mirrors are extremely difficult to sand and polish in order to reach the required standards for space optics and are susceptible to damage by space debris that may compromise the mirror’s quality.
These complicating issues result in the size, durability, and resolution limitations of current space optics technology. As space exploration demands more agile and efficient instruments, researchers at NASA have turned their attention to the use of liquid mirrors as a potential alternative.
Why liquid?
Liquid mirrors in space offer the potential for much larger reflective surfaces, a critical factor in enhancing light-gathering capabilities and improving overall telescope performance. Unlike rigid solid mirrors, liquid mirrors can adapt their shape, allowing for the creation of significantly larger parabolic surfaces in microgravity.
The Fluidic Telescope (FLUTE) project team, jointly led by NASA and Technion – Israel Institute of Technology, led experiments in zero gravity on parabolic flights – aircraft maneuvers that create brief periods of weightlessness to simulate a microgravity environment – and aboard the International Space Station where liquids were injected in a circular frame, resulting in a free-standing liquid lens held together by the surface tension of the liquid. These experiments demonstrated the conceptual basis for the deployment of large fluidic mirrors in space with a large enough circular frame and volume of liquid mirror material.
The adaptability of the mirror would not only facilitate the construction of expansive mirrors of a proposed diameter of 50 meters, but would also ensure impeccable smoothness in the reflective surface, mitigating imperfections that can hinder image quality. Additionally, the dynamic nature of liquid mirrors introduces the intriguing possibility of self-healing properties, where any damage sustained by the mirror can mend itself without requiring any maintenance.
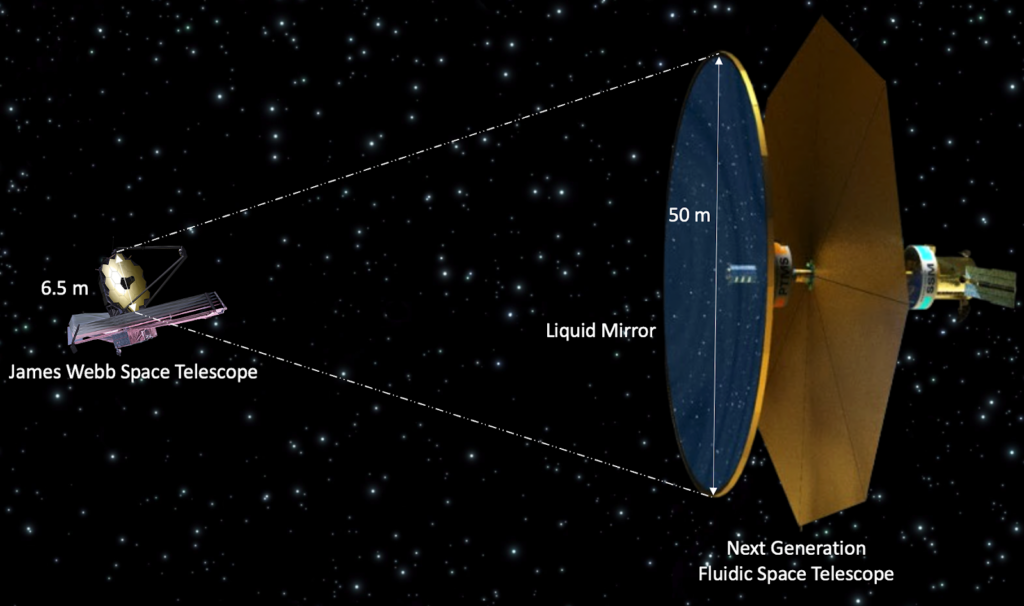
How it’s possible
My time as an intern at NASA’s Goddard Space Flight Center from June 2023 to May 2024 has been dedicated to experimentally developing and testing formulations that could potentially be used for this space-compatible liquid mirror material, which poses several intricate challenges.
One primary concern is identifying a substance that is stable in vacuum without boiling and remains a liquid in the harsh cold conditions of outer space. To address these challenges, ionic liquids were selected as the basis for the liquid mirror technology. Ionic liquids are a class of chemical compounds that are composed entirely of ions, have extremely low melting points, and in lots of cases almost negligible vapor pressures, meaning that they do not boil in vacuum as most liquids would. However, other components also need to be introduced to the ionic liquid to make it reflective like a mirror. To accomplish this, in my experiments I coated ionic liquids with extremely small silver particles, called nanoparticles, to obtain a mirroring fluid that was stable as a liquid in vacuum conditions.
How was this accomplished? Well, a formulation was developed which included a silver-containing molecule (such as AgNO3 or AgBF4) dissolved in the ionic liquid along with a photoinitiator, which is a molecule that becomes reactive once exposed to certain wavelengths of light. Once the silver-containing molecules are dissolved in the liquid resulting in free floating silver ions (Ag+), UV light is applied to the sample. The photoinitiator then absorbs this light and reacts with the silver ions to produce pure silver nanoparticles. These particles are most concentrated on the surface of the liquid, since those regions receive the most UV light. As a result, a smooth, reflective coat of silver is produced on the surface of the ionic liquid.
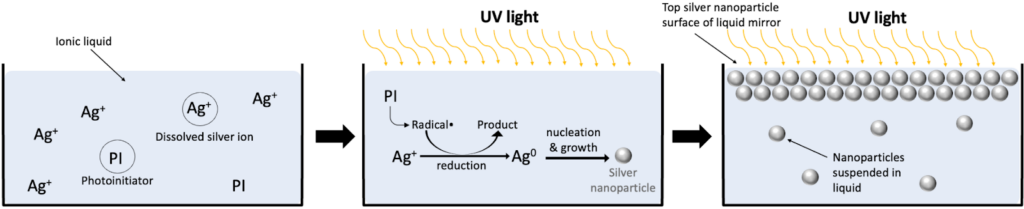
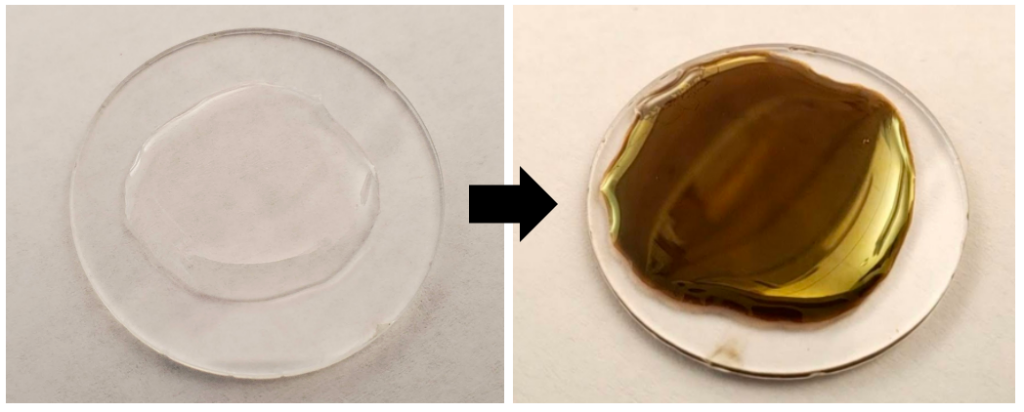
This methodology offers a direct way to transform a space-compatible liquid into a mirror that is stable in vacuum by simply exposing it to UV light. Another benefit of this method is that if the surface coating is damaged, additional UV exposure will heal the surface by causing the formation of new nanoparticles that fill in the damaged portion of the surface.
Looking to the future!
The next steps in this research is to further optimize the formulation to increase the reflective capabilities and robustness of the liquid, test the liquid mirror in microgravity to observe its behavior, and do an in-depth characterization of the surface coating to determine things like the density and thickness of the silver coating.
The hope is that this work can be the foundation in the utilization of liquid mirrors in space telescopes, which could revolutionize astronomical observation by offering larger mirrors, smoother surfaces, and the potential for self-healing capabilities, ushering in a new era of precision and clarity in our exploration of the cosmos.
Titus Szobody is an undergraduate student at North Carolina State University studying chemical and biomolecular engineering. Szobody interned at NASA’s Goddard Space Flight Center for multiple semesters and received funding from NC Space Grant for this work. Szobody will be presenting his research at the 2024 NC Space Symposium.
This post was originally published in NC Space Grant News.